URL: https://www.desy.de/news/news_search/index_eng.html
Breadcrumb Navigation
DESY News: Quantum Physics in Proteins
News
News from the DESY research centre
Quantum Physics in Proteins
A new analytical technique is able to provide hitherto unattainable insights into the extremely rapid dynamics of biomolecules. The team of developers, led by Abbas Ourmazd from the University of Wisconsin–Milwaukee and Robin Santra from DESY, is presenting its clever combination of quantum physics and molecular biology in the scientific journal Nature. The scientists used the technique to track the way in which the photoactive yellow protein (PYP) undergoes changes in its structure in less than a trillionth of a second after being excited by light.
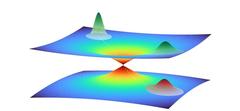
Illustration of a quantum wave packet in close vicinity of a conical intersection between two potential energy surfaces. The wave packet represents the collective motion of multiple atoms in the photoactive yellow protein. A part of the wave packet moves through the intersection from one potential energy surface to the other, while the another part remains on the top surface, leading to a superposition of quantum states. Credit: DESY, Niels Breckwoldt
Whereas a hand is large enough and the movement is slow enough for us to follow it with our eyes, things are not that easy when looking at molecules. The energy state of a molecule can be determined with great precision using spectroscopy; and bright X-rays for example from an X-ray laser can be used to analyse the shape of a molecule. The extremely short wavelength of X-rays means that they can resolve very small spatial structures, such as the positions of the atoms within a molecule. However, the result is not an image like a photograph, but instead a characteristic interference pattern, which can be used to deduce the spatial structure that created it.
Bright and short X-ray flashes
Since the movements are extremely rapid at the molecular level, the scientists have to use extremely short X-ray pulses to prevent the image from being blurred. It was only with the advent of X-ray lasers that it became possible to produce sufficiently bright and short X-ray pulses to capture these dynamics. However, since molecular dynamics takes place in the realm of quantum physics where the laws of physics deviate from our everyday experience, the measurements can only be interpreted with the help of a quantum-physical analysis.
A peculiar feature of photoactive proteins needs to be taken into consideration: the incident light excites their electron shell to enter a higher quantum state, and this causes an initial change in the shape of the molecule. This change in shape can in turn result in the excited and ground quantum states overlapping each other. In the resulting quantum jump, the excited state reverts to the ground state, whereby the shape of the molecule initially remains unchanged. The conical intersection between the quantum states therefore opens a pathway to a new spatial structure of the protein in the quantum mechanical ground state.
The team led by Santra and Ourmazd has now succeeded for the first time in unravelling the structural dynamics of a photoactive protein at such a conical intersection. They did so by drawing on machine learning because a full description of the dynamics would in fact require every possible movement of all the particles involved to be considered. This quickly leads to unmanageable equations that cannot be solved.
6000 dimensions
“The photoactive yellow protein we studied consists of some 2000 atoms,” explains Santra, who is a Lead Scientist at DESY and a professor of physics at Universität Hamburg. “Since every atom is basically free to move in all three spatial dimensions, there are a total of 6000 options for movement. That leads to a quantum mechanical equation with 6000 dimensions – which even the most powerful computers today are unable to solve.”
However, computer analyses based on machine learning were able to identify patterns in the collective movement of the atoms in the complex molecule. “It’s like when a hand moves: there, too, we don’t look at each atom individually, but at their collective movement,” explains Santra. Unlike a hand, where the possibilities for collective movement are obvious, these options are not as easy to identify in the atoms of a molecule. However, using this technique, the computer was able to reduce the approximately 6000 dimensions to four. By demonstrating this new method, Santra’s team was also able to characterise a conical intersection of quantum states in a complex molecule made up of thousands of atoms for the first time.
The detailed calculation shows how this conical intersection forms in four-dimensional space and how the photoactive yellow protein drops through it back to its initial state after being excited by light. The scientists can now describe this process in steps of a few dozen femtoseconds (quadrillionths of a second) and thus advance the understanding of photoactive processes. “As a result, quantum physics is providing new insights into a biological system, and biology is providing new ideas for quantum mechanical methodology,” says Santra, who is also a member of the Hamburg Cluster of Excellence “CUI: Advanced Imaging of Matter”. “The two fields are cross-fertilising each other in the process.”
Reference:
Few-fs resolution of a photoactive protein traversing a conical intersection (view article); A. Hosseinizadeh, N. Breckwoldt, R. Fung, R. Sepehr, M. Schmidt, P. Schwander, R. Santra, A. Ourmazd; Nature, 2021; DOI: 10.1038/s41586-021-04050-9